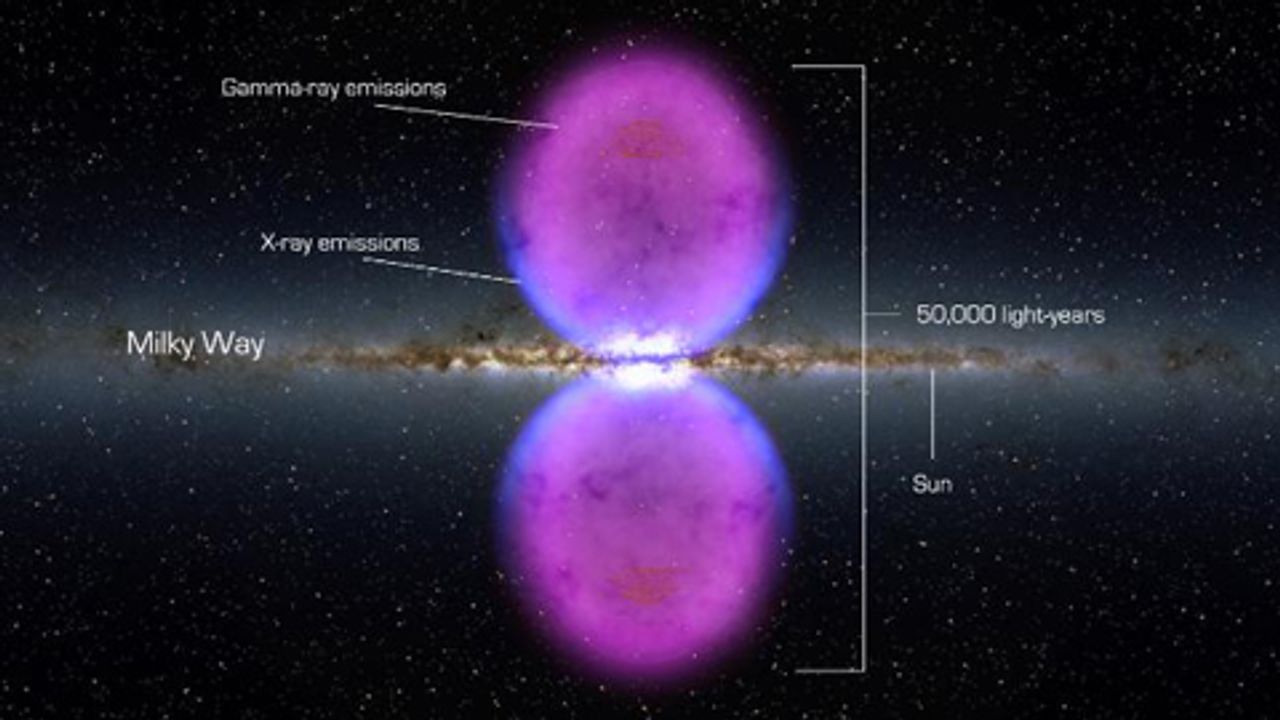
A giant structure around our Milky Way galaxy has been discovered by the NASA Fermi Gamma-ray Space Telescope [1]. Gamma ray emitting bubbles are symmetrically attached at either side of the plane of the Milky Way and are 50,000 light years, or half the Milky Way’s diameter across (one light year is the distance travelled by light in one year, or about 6 trillion miles). Gamma rays, like radio, microwaves, light and X-rays, are electromagnetic radiation travelling at the speed of light but with a much higher energy or shorter wavelength.
Douglas Finkbeiner, an astronomer at the Harvard-Smithsonian Center for Astrophysics, who first discovered the structure, told reporters, “What we see are two gamma ray emitting bubbles that extend 25,000 light years north and south of the galactic centre.” He added, “We don’t fully understand their nature or origin.”
Finkbeiner’s team were on the lookout for something because X-ray observations carried out by the German-led Roentgen satellite in the 1990s had detected hints of the bubbles’ edges close to the galactic centre, shown in blue on the image. Also NASA’s Wilkinson Microwave Anisotropy Probe (WMAP) showed an excess of radio signals in the region of the bubbles.
Nevertheless, this is a totally unexpected discovery. Gamma rays appear as a diffuse fog throughout the sky, as particles moving near to the speed of light interact with the light and interstellar gas in our galaxy. The high-resolution Large Area Telescope (LAT) on Fermi, the most sensitive and highest resolution instrument yet available, was able to detect the bubbles through the fog. The bubbles have a higher energy than the gamma-ray fog and have well-defined edges, suggesting they were caused by a large and relatively rapid release of energy.
Observations of other galaxies have detected jets of plasma ejected from their centre, presumed to be a supermassive black hole. Such jets are believed to emit gamma rays, so one possibility is that the bubbles may be remnants of an eruption from a massive black hole at the centre of our galaxy. Alternatively the bubbles could be the result of gas outflows from a burst of stars that formed in clusters at the galaxy’s centre millions of years ago.
The Fermi Gamma-ray telescope was launched in 2008 and circles the earth in a low orbit. Its main instrument, the LAT, surveys the whole sky every three hours for astrophysical phenomena emitting gamma rays.
Fermi also carries another instrument called the Gamma-ray Burst Monitor (GBM) that studies gamma-ray bursts, of durations from milliseconds to a few minutes, emitted from very distant galaxies. This tells us more about the earlier phases of the universe (the larger the distance away, the longer the time the radiation takes to reach the earth).
Such a new discovery highlights the exciting developments still being made in the science of cosmology, the study of how our universe evolves, and in the related science of astronomy on a vast galactic scale. Together with Fermi, a range of telescopes, both satellite and earthbound, are mapping out the universe in increasing detail in all sectors of the electromagnetic spectrum, from gamma rays through to radio.
It is now well established that our universe evolved from a “big bang” some 13.7 billion years ago [2]. The nature of the big bang and of what came before it are much disputed questions with as yet no evidence to distinguish between competing theories. In the first few microseconds, the universe is understood to have gone through an “inflation” phase, leading to an extremely hot soup of quarks, the nature of which is understood from particle physics and accelerators such as the large hadron collider (LHC) at CERN.
It is now well understood, and backed by observations such as WMAP, that during the first 100 million years, as the quark soup cooled down, space was largely featureless and only about 4.5 percent of it was the kind of matter—protons, neutrons, etc.—of which we are made. A further 23 percent is known to be “dark matter” which interacts through gravity with ordinary matter—it holds together much of the galaxies we observe—but otherwise interacts only very weakly. How the quark phase gave rise to dark matter is a key issue in particle physics.
Originally, dark matter was thought to be made up of similar matter to ours, so-called Massive Astrophysical Compact Halo Objects (MACHOs), but the evidence is now against this and in favour of the whimsically named Weakly Interacting Massive Particles (WIMPs). Such particles, given the label neutralinos, imply an extension of the standard model of particle physics. Another candidate for dark matter is a particle called the axion. Experiments conducted deep beneath the earth’s surface have so far failed to detect dark matter particles passing through. It is also possible that WIMPs will be detected at the LHC at CERN.
The remaining 72 percent of the matter in the universe is made up of “dark energy,” postulated to explain the known acceleration in the expansion of the universe. Its nature is still regarded as a mystery by most scientists [3].
Computer models show that after the first 100 million years or so, slight fluctuations in the thinly spread matter would lead to regions that became denser under the effect of gravity. Such clouds, with the mass of perhaps a million times that of our sun, would be mainly dark matter. But the ordinary part of matter, which by then would have formed atoms of hydrogen or helium, would emit light and become concentrated at the centre of the cloud. Fairly rapidly, these early type of stars—hundreds of times more massive than our current stars—exploded, forming heavier atomic elements, and giving rise to clouds that over the next billion years or so formed under gravity the galaxies that we now observe.
Much remains to be understood about this process of galaxy formation in the early universe. An aspect of astronomy that is now very much researched is the science of “black holes” [4]. It is known that black holes—regions where matter that has become so dense that gravity prevents anything from escaping, including light—are predicted to exist by Einstein’s theory of gravitation. Such objects can be indirectly observed by their effect on nearby matter. They tend to come in two varieties: those with a mass of five to 15 times the mass of our sun, which are the remnants of stars that have collapsed under their own gravity as they have burnt out, and supermassive black holes that are as massive as millions or even billions of suns and exist at the centre of galaxies.
How these supermassive black holes came into being in the early period of the universe, and how they are associated with galaxy formation, is still an open question, with various scenarios being put forward [5]. It is thought that Sagittarius A*, with a mass of 4.5 million suns, is such a black hole at the centre of our Milky Way galaxy.
The difficulty of explaining the existence of supermassive black holes comprised of ordinary matter is that there would not be enough time for them to form in the billion years or so that current observations suggest. One attractive possibility, put forward by Professor Katherine Freese of the University of Michigan at Ann Arbor, is that regions of WIMP dark matter would cool and shrink, producing the first “dark stars”. Such stars could then have collapsed further into supermassive black holes of the size now observed and this could have occurred within a billion years from the big bang, according to Freese and her colleagues [6].
The Fermi space telescope was carrying out a search for gamma rays that are predicted to be produced by collisions within the dark matter surrounding our galaxy. But such gamma rays would be in the form of a fuzzy fog and not a structure like the bubbles. “I don’t think this is dark matter,” said Finkbeiner. “In fact it makes it harder to find dark matter, because now we have this other thing in the way. It just confuses everything.” However he did think that the bubbles could have been caused by the Milky Way’s central black hole. “This might be the first evidence for an outburst of activity of the black hole at the centre of the galaxy,” he said.
Whatever understanding is final arrived at of the gamma-ray bubbles and the overall development of our universe, scientific cosmology fully supports a philosophically materialist outlook. Matter on a galactic scale has its own unique qualitative features, and although different from biological evolution, the evolution of human society, and even the evolution of stars, it has its own specific historical laws that are now being uncovered.
[1] http://www.nasa.gov/mission_pages/GLAST/news/new-structure.html
[2] The universe, Michael S. Turner, Scientific American, September 2009.
[3] Is dark energy really a mystery? Cosmology Forum, Nature, Vol 466, 15 July 2010.
[4] Portrait of a black hole, Avery E. Broderick and Abraham Loeb, Scientific American,
December 2009.
[5] Formation of supermassive black holes, Marta Volonteri, Astronomy and Astrophysics Review,
18, pp 279-315, 2010.
[6] Dark side of black holes, Charles Q. Choi, Scientific American, March 2010.